Beyond the central dogma: DNA assemblies programmed to function as nanoscale motors Restricted; Files & ToC
Bazrafshan, Alisina (Spring 2021)
Abstract
Biological motor proteins that convert chemical energy into controlled nanomechanical motion are essential and shared among all branches of life. For example, groups of myosin motors proteins collectively take defined linear steps to power muscle contraction and vesicle transport. Accordingly, there is considerable interest in developing synthetic analogues. Among the synthetic motors created thus far, unipedal and bipedal DNA walkers that undertake discrete steps on RNA tracks have shown great promise with applications spanning from biosensing to cargo transport. Nonetheless, DNA-based walkers fall short because of their limited speed, low endurance, and the lack of preferred directionality which is ultimately due to the lack of coordination between individual DNA legs. The goal of this thesis is to study how DNA assemblies and nanostructures can be programmed to function as efficient nanoscale motors, and how they can serve as a new platform for chemical sensing that operate far-from-equilibrium. In Chapter 1, we describe the historical context and summarize the representative advances in structural and dynamic DNA nanotechnology. The Chapter also includes a classification of DNA machines and nanodevices, figures of merit for nanoscale motors, and energetic concepts in designing DNA based motors. Chapter 2 reports on the development of the first example of a DNA origami nanostructure that is programmed to function as a nanoscale motor. The DNA origami is a 16 helix-bundle structure that is coated with DNA ‘legs’. DNA leg’s bind to a surface coated with RNA footholds and upon
addition of RNase H will function as a motor, burning RNA fuel and rolling along the RNA track to bind fresh RNA. Because DNA origami enables precise testing of nanoscale structure-function relationships, we were able to experimentally study the role of motor shape, chassis flexibility, leg distribution, and total number of legs in tuning performance. An anisotropic rigid chassis coupled with a high density of legs maximizes nanomotor speed and endurance. DNA origami motors travel micron distances at an average speed of 0.6 nm/s unidirectionally without intervention through an external force field or patterned tracks.
In Chapter 3, we expanded upon these studies and used a combination of modelling and experiments to investigate whether we could further enhance the performance of synthetic nanoscale DNA motors. We used DNA-gold nanoparticle conjugates and tuned structural parameters such as DNA leg density, leg span, and nanoparticle anisotropy as well as buffer conditions to enhance motor performance. Both modeling and experiments demonstrate that increasing DNA leg density boosts the speed and processivity of motors, whereas DNA leg span increases processivity. By taking advantage of label-free imaging of nanomotors, we also uncover Lévy-type motion where motors exhibit bursts of translocation that are punctuated with transient stalling. Dimerized particles also demonstrate more ballistic trajectories confirming a rolling mechanism. Our work shows the fundamental properties that control DNA motor performance and demonstrates enhanced motors that can travel multiple microns within minutes with velocities of up to 50 nm/s. The performance of these nanoscale motors approaches that of motor proteins that travel at speeds of 100-1000 nm/s, and hence this work can be important in developing proto-cellular systems as well next generation sensors and diagnostics.
Chapter 4 describes the application of these motors as nanoscale sensors. DNA based motors offer a unique sensing platform since the motion of these motors is exquisitely sensitive to the molecular interactions experienced by the motor. In this Chapter, we aimed to find out whether DNA hybridization could act as a molecular “brake” to stop or slow down the motion of our developed motors. We find that sensitivity of DNA based motors has a negative correlation with size. We demonstrate that using 50 nm DNA motors we can sense multiple transient interactions or single DNA duplexes.
Finally, Chapter 5 provides a perspective discussing other strategies to design DNA based nanoscale motors that efficiently convert chemical energy to nanomechanical motion. I will summarize our efforts to alter the chemical reaction used to power nanoscale DNA motors as well as alternative track designs to construct nanoscale DNA based motor systems. Such constructs are anticipated to have emergent properties that impact biosensing and gene editing.
Table of Contents
This table of contents is under embargo until 25 May 2027
About this Dissertation
School | |
---|---|
Department | |
Degree | |
Submission | |
Language |
|
Research Field | |
Palavra-chave | |
Committee Chair / Thesis Advisor | |
Committee Members |
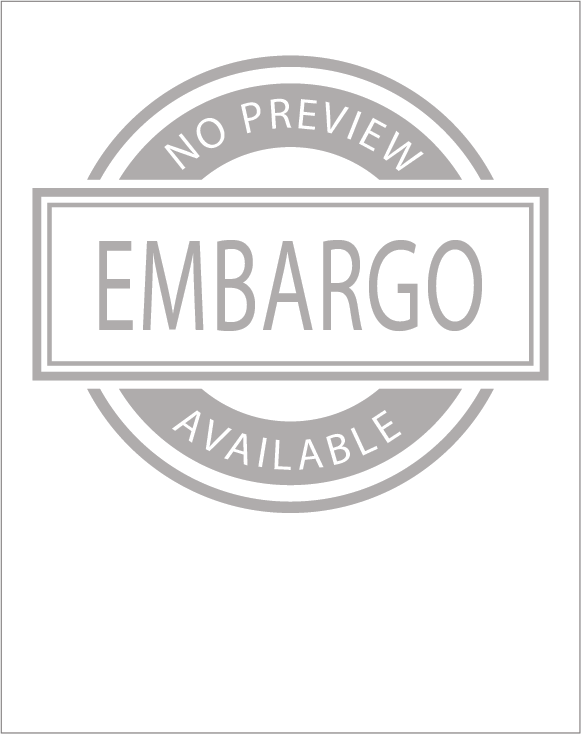
Primary PDF
Thumbnail | Title | Date Uploaded | Actions |
---|---|---|---|
![]() |
File download under embargo until 25 May 2027 | 2021-05-10 09:05:33 -0400 | File download under embargo until 25 May 2027 |
Supplemental Files
Thumbnail | Title | Date Uploaded | Actions |
---|---|---|---|
![]() |
File download under embargo until 25 May 2027 | 2021-05-10 09:05:46 -0400 | File download under embargo until 25 May 2027 |
![]() |
File download under embargo until 25 May 2027 | 2021-05-10 09:05:53 -0400 | File download under embargo until 25 May 2027 |